By Paul Nicolaus
September 30, 2020 | New research offers a glimpse into the future of wearables, which may use everyday tools like pens, pencils, and paper to draw monitoring devices directly onto skin.
Currently, wearables often come in the form of smart watches, activity trackers, or soft patches that stick to the skin. While they have become a useful way to monitor, prevent, or treat illness and injury by tracking physiological information, even the most flexible wearable sensors do not move fully with the skin.
If a fitness tracker reads 8,500 steps rather than, say, 8,600 over the course of a day, this type of imprecision may not be a big deal. But sensors meant to track heart function or other bodily signals need to be accurate if they are going to be used for diagnostics and treatment.
Adhesion or conformability issues could lead to misinterpretation and misdiagnoses, according to a team of researchers from the University of Houston and the University of Chicago, who revealed a new drawn-on-skin bioelectronics platform in a paper published July 30 in Nature Communications (doi: 10.1038/s41467-020-17619-1).
“The problem facing us and also the whole field is the motion artifact,” Cunjiang Yu, associate professor of mechanical engineering at the University of Houston, told Diagnostics World. When people move around, the signal quality worsens. Reducing or completely removing this motion artifact is a huge challenge, he said.
The group’s recently published research, which is supported by the Office of Naval Research and National Institutes of Health, tackles this challenge.
“Drawn-on-Skin” Electronics
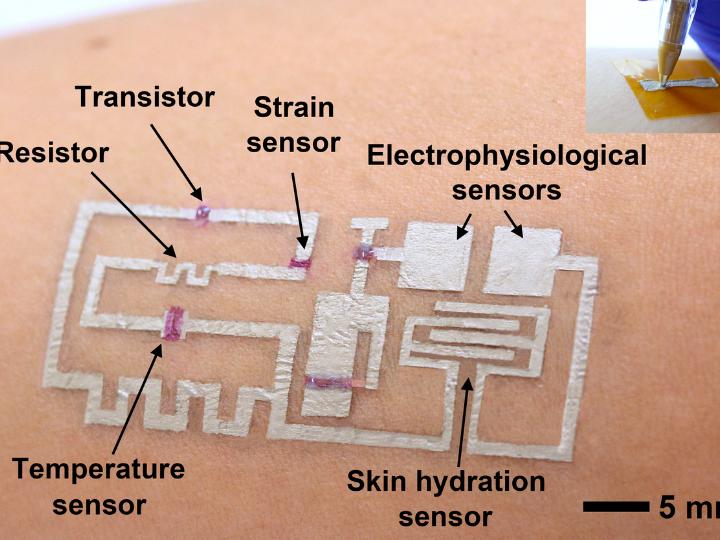
According to the researchers, their work addresses the problem of gathering accurate biological data while a person is in motion by drawing sensors and circuits onto skin.
The original ink is removed from a ballpoint pen and filled with electronic liquid ink—including conductors, semiconductors, and dielectrics—that are then drawn on the skin directly with or without a stencil.
One component of the conductor ink precursor is silver nanoparticle, Yu explained. Some circuits only require the use of one ink material i.e. conductors, semiconductors, and dielectrics whereas other circuits call for more than one material delivered by multiple pens.
The application is similar to the way anyone would use a pen to write on a piece of paper or scrawl a phone number on the back of your hand. And according to Yu, anyone could handle the drawing process—not just medical professionals.
Coming out of the pen, the electronic materials are liquid, and like ink on paper, the electronics dry quickly. According to the study, after about five minutes of evaporation at room temperature, the stencil was removed, and a dry, thin film remained on the skin. Any imperfections in the drawn-on-skin device can be corrected by drawing over them. The technology is capable of tracking physical data such as muscle signals, heart rate, temperature, and skin hydration.
The interface between the electronic and the skin is very stable, Yu explained, making it possible to gather more precise data and transmit that information with wires or wirelessly.
The wireless monitoring system, for instance, included an amplifier, microcontroller, Bluetooth module, and 3.7 volt lithium-ion polymer battery. The amplifier's electrical leads were put onto a conductive tape laminated onto the drawn-on-skin sensors, and the microcontroller captured data via Bluetooth and displayed that information in real-time.
The technology offers several advantages over existing wearables. “First of all, you don’t need to create a traditional wearable device,” Yu said. With this drawn-on-skin technology, you just need a pen or pens to form a circuit, which means it is both easy and low-cost. The device is relatively unaffected by sweat and can be worn while taking a shower, Yu noted, and if the device becomes damaged it is possible to repair the circuit.
The technology could be particularly useful in low resource areas, according to the researchers. And in an entirely different application, there is potential to speed along the healing process for skin wounds using pulsed electrical stimulation. Drawn-on-skin electronics can flex to fit an injury’s unique shape and size, which may be useful to soldiers in battlefield settings.
To demonstrate the wound healing abilities, the research group created skin wounds on the back of mice and drew electrodes on half of those injuries, leaving the other half untreated. Histology images revealed that the treated portion showed only a thin layer of scab, while the untreated half had a large scab. Images also revealed that the cross-section of the treated portion had a narrower wound width. By day five, the untreated scab width had shrunk from 1cm to .49cm and the treated scab from 1cm to .20cm, demonstrating accelerated wound recovery.
Because the simple drawing process makes it possible for people of any background or knowledge level to create drawn-on-skin electronics, the researchers believe further development and optimization could ultimately result in a new, easy, and accessible healthcare tool.
Duncan McClure, a biomedical engineer who was not involved in the study, said the work presented by Yu and colleagues is significant in the sense that it demonstrates drawn-on-skin electronics that function in standard conditions is possible.
“I think the diagnostic implications of such a draw-to-skin circuit would be the potential for very low-cost diagnostics, especially in resource-poor areas, assuming usable results can be obtained easily,” he told Diagnostics World. Compared to the wearable biosensors that he is aware of—either on the market or reported in scientific literature—he sees several potential upsides and drawbacks.
Among the upsides is the ease of application considering someone with minimal training could use a special pen to draw a circuit using the stencil. He highlighted the possible reduction in production and distribution costs, too, considering the inks used can be packed into a pen that can store enough to draw tens or maybe even hundreds of circuits.
“This is significant because most other skin biosensor methods I have read about require printing onto blotter paper or a band-aid-like device,” McClure explained, which leads to additional material and manufacturing costs and often requires individual packaging. These savings can potentially be passed along to the end customer.
In terms of potential drawbacks, nanoparticle sensors tend to require tight tolerances for the amount deposited and the layer thickness to work, he noted, and there appears to be no guarantee of either when using a pen to stencil a circuit onto the skin. “I would be concerned too much or too little ink may adversely affect the sensor’s output,” he added.
Although he acknowledged that he has no special expertise in wound care, McClure also voiced concern about the potential for infection if this technology were to be used for wound healing purposes in particular.
Using Pencil and Paper
In a similar endeavor, researchers at the University of Missouri, University of Illinois-Chicago, University of Illinois Urbana-Champaign, and Yale University have demonstrated that pencils and paper could be used to monitor personal health in a study published in the journal Proceedings of the National Academy of Sciences (doi: 10.1073/pnas.2008422117). One day, according to their vision, people could simply pick up a pencil and draw a bioelectronic device onto their skin.
They discovered that pencils with more than 90% graphite could conduct a high amount of energy generated by the friction between paper and pencil. More specifically, they found that pencils with 93% graphite were ideal for creating an array of bioelectronic devices drawn on commercial office copy paper. A biocompatible spray-on adhesive can then be applied to help the paper stick to an individual’s skin.
In terms of diagnostic potential, the study—supported by a University of Missouri start-up fund and grants from the National Science Foundation, Air Force Office of Scientific Research, and National Institutes of Health—reveals that pencil and paper bioelectronics are capable of monitoring electrophysiological signals, temperature, and chemicals in sweat.
In the paper, the researchers report a variety of pencil and paper-based on-skin electronic devices, including biophysical sensors, sweat biochemical sensors, thermal stimulators, and humidity energy harvesters. Pencil-drawn graphite patterns serve as conductive traces and sensing electrodes, and office-copy papers serve as flexible supporting substrates. The devices can perform real-time, continuous monitoring of skin temperatures, electrocardiograms, electromyograms, instantaneous heart rates, respiratory rates, as well as sweat pH, uric acid, and glucose.
According to Zheng Yan, an assistant professor at the University of Missouri College of Engineering, electrophysiological signals are strongly correlated with people’s health status. EEGs can provide insights into normal brain function and neurological disorders such as Parkinson’s disease, epilepsy, stroke, and restless leg syndrome, he told Diagnostics World. ECGs can offer insights into normal cardiac function and dysfunctions like arrhythmias, and EMGs can help identify neuromuscular diseases.
Sweat analysis is currently used for applications like disease diagnosis, drug abuse detection, and the optimization of athletic performance, Yan added. Sweat glucose concentration is an indicator of blood glucose level, which is important for diabetes management. Uric acid is a risk factor for conditions such as cardiovascular disease, type 2 diabetes, and renal disease, and it has been used in clinical settings to manage gout. And variations in skin pH levels are a sign of skin disorders such as dermatitis and fungal infections.
Many existing on-skin biomedical devices tend to include two main components, he explained. There is a biomedical tracking component and a surrounding material that provides a supportive structure for that component to maintain an on-skin connection with the body. But this approach is usually complicated and expensive to produce.
Compared to existing fabrication techniques, pencil and paper-based on-skin bioelectronics are appealing for a number of reasons, including the wide availability of fabrication materials, simple fabrication process, low cost, and disposability. Paper’s flexibility and breathability can also benefit its on-skin electronics applications.
Yet another advantage is that paper can decompose in a matter of weeks, according to the researchers, whereas many commercial devices include parts that are not easily broken down. Most polymer substrates in on-skin electronics require hundreds of years to decompose in natural environments, for instance.
These pencil and paper bioelectronics can potentially find applications in low-resource environments such as developing countries or combat fields as well as home-based, personalized health care. They could also be utilized within hospital settings for the real-time, continuous recording of patients’ physiological signals. Beyond that, the approach may enable scientists to carry out remote research.
Next Steps
Both University of Houston’s Yu and University of Missouri’s Yan noted that there are still many challenges that need to be addressed.
By Yu’s rough estimations, his group’s new form of wearable electronics is probably still two or three years away from utilization in real-world settings. Moving forward, he and his colleagues are looking to extend the life of these wearables. (Currently, the ink-on-skin devices can last up to a week, he said.)
Meanwhile, Yan indicated that research exploring the use of pencil and paper on-skin bioelectronics is still in its infancy. He and colleagues plan to further develop and test the use of the biomedical components, including biochemical, temperature, and electrophysiological sensors.
Office-copy paper is not comfortable to wear, he pointed out, and while the direct pencil drawing of bioelectronics onto papers is simple, it is not yet scalable. His research group intends to explore chemical treatment methods to improve the comfort level of paper-based bioelectronics and pursue more scalable fabrication approaches for the mass production of these inexpensive and biodegradable devices.
Paul Nicolaus is a freelance writer specializing in science, nature, and health. Learn more at www.nicolauswriting.com.